Tubular extensions of plant organelles and their implications on retrograde signaling
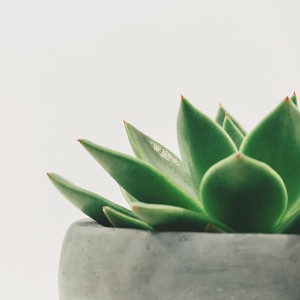
Accepted: November 13, 2023
All claims expressed in this article are solely those of the authors and do not necessarily represent those of their affiliated organizations, or those of the publisher, the editors and the reviewers. Any product that may be evaluated in this article or claim that may be made by its manufacturer is not guaranteed or endorsed by the publisher.
Authors
Tubular extensions emerging from plastids, termed stromules, have received renewed attention due to advancements in imaging techniques. Stromules are widespread in plant and algal species; however, their role in organelle communication and physiology is yet to be elucidated. Initially, stromules were thought to facilitate interplastid communication; however, this proposition is still debated. Stromules with diameters of 0.3-0.8 µm enable protein movement via diffusion and Adenosine Triphosphate (ATP)-dependent transport. Stromule formation is more evident in non-photosynthetic plastids and is induced by various biotic and abiotic stresses, suggesting the involvement of stress-triggered signal transduction via phytohormones and redox changes. Recent studies have emphasized the significance of stromules in plant immunity, especially in response to viral and bacterial effectors, where they serve as conduits for the transport of retrograde signaling molecules from the plastids to the nucleus. Peroxules and matrixules, extending from peroxisomes and mitochondria, respectively, are parallel tubular extensions that were originally found in plant cells, while similar structures also exist in mammalian cells. The response of these extensions to stress may contribute to the management of Reactive Oxygen Species (ROS) and organelle proliferation. This short review discusses the potential roles of the organelle extensions in retrograde signaling pathways.
How to Cite

This work is licensed under a Creative Commons Attribution-NonCommercial 4.0 International License.
PAGEPress has chosen to apply the Creative Commons Attribution NonCommercial 4.0 International License (CC BY-NC 4.0) to all manuscripts to be published.