Muscle hypertrophy and muscle strength: dependent or independent variables? A provocative review
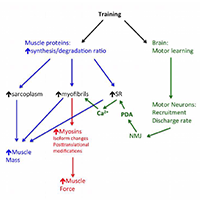
Submitted: 17 August 2020
Accepted: 23 August 2020
Published: 9 September 2020
Accepted: 23 August 2020
Abstract Views: 7981
PDF: 4027
HTML: 112
HTML: 112
Publisher's note
All claims expressed in this article are solely those of the authors and do not necessarily represent those of their affiliated organizations, or those of the publisher, the editors and the reviewers. Any product that may be evaluated in this article or claim that may be made by its manufacturer is not guaranteed or endorsed by the publisher.
All claims expressed in this article are solely those of the authors and do not necessarily represent those of their affiliated organizations, or those of the publisher, the editors and the reviewers. Any product that may be evaluated in this article or claim that may be made by its manufacturer is not guaranteed or endorsed by the publisher.
Similar Articles
- Sandra Zampieri, Ines Bersch, Piera Smeriglio, Elena Barbieri, Simona Boncompagni, Maria Chiara Maccarone, Ugo Carraro, Program with last minute abstracts of the Padua Days on Muscle and Mobility Medicine, 27 February – 2 March, 2024 (2024Pdm3) , European Journal of Translational Myology: Vol. 34 No. 1 (2024)
- Sandra Zampieri, Ines Bersch, Piera Smeriglio, Elena Barbieri, Massimo Ganassi, Christiaan Leeuwenburg, Riccardo Rosati, Paolo Gargiulo, Amber Pond, H. Lee Sweeney, Ugo Carraro, Five Padua days on muscle and mobility medicine (2024Pdm3) 27 February - 2 March, 2024 at Hotel Petrarca, Thermae of Euganean Hills, Padua, and San Luca Hall, Prato della Valle, Padua, Italy , European Journal of Translational Myology: Vol. 33 No. 4 (2023)
- The Editors, Abstracts of the 2016 Spring Padua Muscle Days: Muscle decline in aging and neuromuscular disorders - Mechanisms and countermeasures | Terme Euganee, Padua, Italy, April 13-16, 2016 , European Journal of Translational Myology: Vol. 26 No. 1 (2016)
- The Editors, Abstracts of the 2017 Spring Padua Muscle Days: Translational Myology for Impaired Mobility | Euganei Hills, Padua, Italy, March 23-25, 2017 , European Journal of Translational Myology: Vol. 27 No. 2 (2017)
- Maurilio Sampaolesi, Stefania Fulle, Report and Abstracts of the 19th Meeting of the Interuniversity Institute of Myology: Assisi, October 20-23, 2022 , European Journal of Translational Myology: Vol. 33 No. 2 (2023)
- Ugo Carraro, Exciting perspectives for Translational Myology in the Abstracts of the 2018Spring PaduaMuscleDays: Giovanni Salviati Memorial – Chapter IV - Abstracts of March 17, 2018 , European Journal of Translational Myology: Vol. 28 No. 1 (2018)
- Paul Dowling, Stephen Gargan, Margit Zweyer, Michael Henry, Paula Meleady, Dieter Swandulla, Kay Ohlendieck, Proteomic reference map for sarcopenia research: mass spectrometric identification of key muscle proteins located in the sarcomere, cytoskeleton and the extracellular matrix , European Journal of Translational Myology: Vol. 34 No. 2 (2024)
- Paul Dowling, Stephen Gargan, Margit Zweyer, Michael Henry, Paula Meleady, Dieter Swandulla, Kay Ohlendieck, Proteomic reference map for sarcopenia research: mass spectrometric identification of key muscle proteins of organelles, cellular signaling, bioenergetic metabolism and molecular chaperoning , European Journal of Translational Myology: Vol. 34 No. 2 (2024)
- Sascha Sajer, Mobility disorders and pain, interrelations that need new research concepts and advanced clinical commitments , European Journal of Translational Myology: Vol 27, No 4 (2017)
- Ugo Carraro, Exciting perspectives for Translational Myology in the Abstracts of the 2018Spring PaduaMuscleDays: Giovanni Salviati Memorial – Chapter II - Abstracts of March 15, 2018 , European Journal of Translational Myology: Vol. 28 No. 1 (2018)
You may also start an advanced similarity search for this article.