Increase of resting muscle stiffness, a less considered component of age-related skeletal muscle impairment
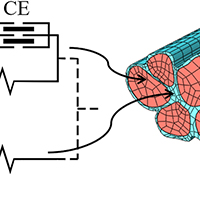
Accepted: 6 April 2020
HTML: 75
All claims expressed in this article are solely those of the authors and do not necessarily represent those of their affiliated organizations, or those of the publisher, the editors and the reviewers. Any product that may be evaluated in this article or claim that may be made by its manufacturer is not guaranteed or endorsed by the publisher.
Authors
Elderly people perform more slowly movements of everyday life as rising from a chair, walking, and climbing stairs. This is in the first place due to the loss of muscle contractile force which is even more pronounced than the loss of muscle mass. In addition, a secondary, but not negligible, component is the rigidity or increased stiffness which requires greater effort to produce the same movement and limits the range of motion of the joints. In this short review, we discuss the possible determinants of the limitations of joint mobility in healthy elderly, starting with the age-dependent alterations of the articular structure and focusing on the increased stiffness of the skeletal muscles. Thereafter, the possible mechanisms of the increased stiffness of the muscle-tendon complex are considered, among them changes in the muscle fibers, alterations of the connective components (extracellular matrix or ECM, aponeurosis, fascia and tendon) and remodeling of the neural pattern of muscle activation with increased of antagonist co-activation.
How to Cite
PAGEPress has chosen to apply the Creative Commons Attribution NonCommercial 4.0 International License (CC BY-NC 4.0) to all manuscripts to be published.
Similar Articles
- Sandra Zampieri, Ines Bersch, Piera Smeriglio, Elena Barbieri, Simona Boncompagni, Maria Chiara Maccarone, Ugo Carraro, Program with last minute abstracts of the Padua Days on Muscle and Mobility Medicine, 27 February – 2 March, 2024 (2024Pdm3) , European Journal of Translational Myology: Vol. 34 No. 1 (2024)
- Ugo Carraro, Exciting perspectives for Translational Myology in the Abstracts of the 2018Spring PaduaMuscleDays: Giovanni Salviati Memorial – Chapter IV - Abstracts of March 17, 2018 , European Journal of Translational Myology: Vol. 28 No. 1 (2018)
- Sandra Zampieri, Ines Bersch, Piera Smeriglio, Elena Barbieri, Massimo Ganassi, Christiaan Leeuwenburg, Riccardo Rosati, Paolo Gargiulo, Amber Pond, H. Lee Sweeney, Ugo Carraro, Five Padua days on muscle and mobility medicine (2024Pdm3) 27 February - 2 March, 2024 at Hotel Petrarca, Thermae of Euganean Hills, Padua, and San Luca Hall, Prato della Valle, Padua, Italy , European Journal of Translational Myology: Vol. 33 No. 4 (2023)
You may also start an advanced similarity search for this article.